Edge States Drive Exciton Dissociation in Ruddlesden–Popper Lead Halide Perovskite Thin Films
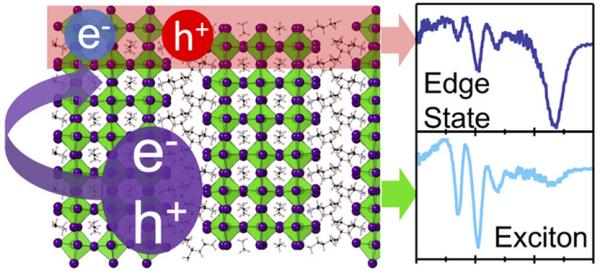
Citation
Kinigstein, E.D.; Tsai, H.; Nie, W.; Blancon, J.-C.; Yager, K.G.; Appavoo, K.; Even, J.; Kanatzidis, M.G.; Mohite, A.D.; Sfeir, M.Y. "Edge States Drive Exciton Dissociation in Ruddlesden–Popper Lead Halide Perovskite Thin Films"
ACS Materials Letters 2020,
2 1360–1367.
doi: 10.1021/acsmaterialslett.0c00333Summary
We investigate electronic states appearing at the surfaces of perovskite thin films.
Abstract
Efficient photovoltaic cells based on thin films of solution-processed 2D Ruddlesden–Popper hybrid perovskites (RPPs) represent an exciting breakthrough due to their enhanced tunability and chemical stability relative to those fabricated from 3D phases. However, reports of efficient charge separation and current collection are in apparent contradiction with the well-known enhancement of the exciton binding energy in multilayered halide perovskites, which should lower the device’s internal quantum efficiency and voltage. This controversy has led to various proposals for the electronic and physical structure of RPP thin films, including phase inhomogeneity as the driving force for exciton dissociation and transport. We address this apparent paradox in high-quality hot-cast RPP films by correlating ultrafast transient absorption spectroscopy with X-ray scattering measurements. We show that a hot-casting fabrication method produces highly phase pure n = 3 (BA)2(MA)n-1PbnI3n+1 RPP structures. The high-phase purity and large grain sizes allow us to observe vertical transport of excitons via a diffusive process and allow us to determine that charge separation is primarily driven by dissociation at surface localized subgap electronic states. We analyze the differential absorption kinetics in films of varying thickness to directly determine that the excitonic diffusion constant is ~0.18 cm^2 s^-1. We propose that a surface localized structural distortion, observed using surface selective grazing incidence X-ray scattering measurements, is responsible for the creation of the surface localized defect states. We find that the density and spatial distribution of these defect states is a function of preparation conditions.