Machine-learning for Science
Since 2011, I have been leading a research program in the application of artificial intelligence and machine-learning (AI/ML) methods to scientific problems, especially x-ray scattering experiments. Through collaborations, we investigated how machine vision and deep learning (convolutional neural network) methods can be used to cluster and classify x-ray scattering detector images. We also demonstrated the application of ML methods to data healing, ptychographic reconstruction, and identification of atomically-thin flakes of 2D materials in optical images.
I was among the pioneers in demonstrating autonomous experimentation, wherein the scientific instrument autonomously guides experiment execution, liberating the human scientist to think about the scientific problem at a higher level. Our research collaboration demonstrated autonomous x-ray scattering at several synchrotron beamlines (at NSLS-II), by combining automated data analysis with a Gaussian Process decision-making algorithm. Ongoing work is pushing these methods towards real-time machine-guided material synthesis.
I am now focused on building a science exocortex, a swarm of AI agents that expand a researcher’s cognitive capabilities. The AI agents provide the user with a streamlined interface to research tasks, coordinate with each other to solve more complex problems, and ultimately enhance the human researcher’s intellectual reach. This is an ambitious plan that will require contributions across the science community.
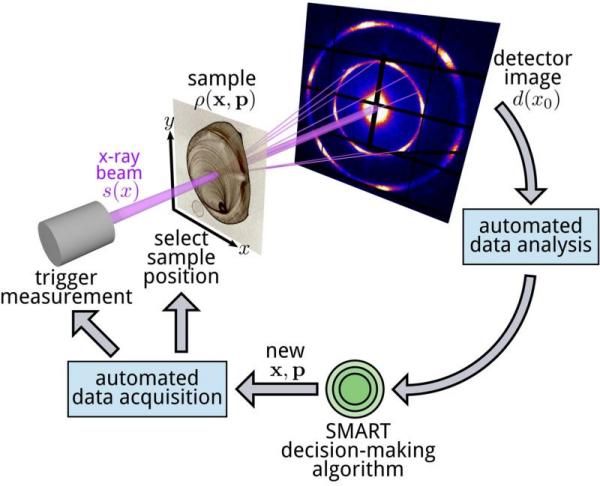
Non-equilibrium Self-Assembly
Self-assembly is canonically thought of as an equilibrium process, where the materials order according to energy-minimization. The important role of non-equilibrium effects is becoming increasingly clear: self-assembly is influenced by kinetic trapping, metastable states, coexisting phases, and process-history dependent order. While this is often viewed as a nuisance (kinetics limiting the quality of order that one can easily achieve), it is also possible to exploit these non-equilibrium effects to control order-formation in self-assembly.
I am working on a suite of methods to control the ordering of block-copolymer thin films, exploiting non-equilibrium effects. For instance, I demonstrated how extreme thermal gradients, generated using photothermal methods, can enhance grain coarsening kinetics, and allow the morphology to be aligned. Moreover, these methods allow one to probe the complex pathway-dependent ordering histories of block-copolymers. More generally, I am interested in demonstrating how contrived arrangements of block-copolymer materials (e.g. layering) can be used to induce the formation of morphologies that do not appear in the native (bulk equilibrium) phase diagram.
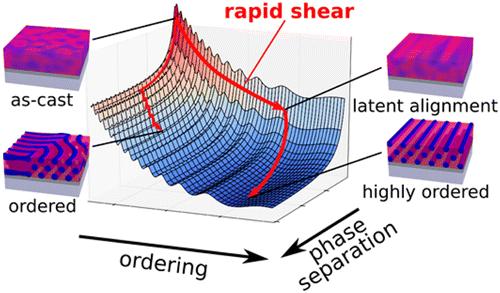
Scattering Measurements
I have longstanding interests in developing scattering measurement methods (using x-rays and neutrons) to more precisely measure nanostructures. I developed a rotational SANS to enable mapping reciprocal-space to characterize 3D order. I co-developed grazing-incidence transmission small-angle x-ray scattering (GTSAXS) as a way to perform GISAXS measurements without the complicating distortions. I developed the mathematical framework and software code for simulating and fitting scattering data from nanoscale superlattices. More recently, I have been interested in variance scattering; that is, analysis methods that exploit correlations within datasets. For instance, I demonstrated how the non-uniformity of scattering rings can be used to calculate grain size. My group also demonstrated how angular correlation analysis can be combined with coherent scattering to enable “amplified” measurements; that is, a mode where scattering signals are boosted above an interfering background by bringing the sample close to a strongly-scattering amplifier. Most recently, I have deployed computational methods for healing x-ray data, including filling gaps in SAXS data, and computationally unwarping GISAXS data.
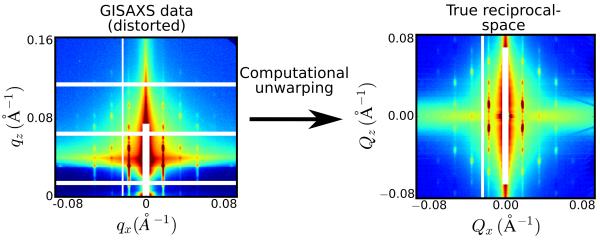
Templated Self-Assembly (Postdoctoral Research)
I worked as a postdoctoral guest researcher at NIST, on problems in templated and directed self-assembly. In particular, I studied how model self-assembling systems (especially block-copolymers) can be influenced by a variety of directing forces, such as topographical templates, rough interfaces, and thermal fields. For instance, I showed that robust biasing of the self-assembly process can be achieved using simple nanoparticle-treated substrates, since these inherently rough surfaces drastically alter the self-assembly energy landscape.
Integral to my work at NIST was the development of measurement techniques and methodologies for characterizing nanostructures. In particular we identified through industrial partners that knowledge of the orientational distribution of structures is a crucial parameter for serious use of self-assembly. For instance, alignment of block-copolymer cylinders can be used as a mask for subsequent etching, enabling production of extremely small-scale devices. The angular distribution of the cylinder axes plays a critical role in determining the quality of any pattern transfer, yet many techniques (e.g. AFM) can only probe the surface order. I thus been developed scattering techniques (rotational SANS, GISAXS, off-specular reflectivity, etc.) in order to quantitatively determine the orientational order in these systems.
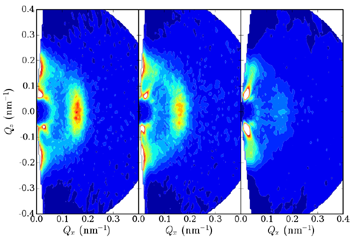
Photoresponsive Polymers (Ph.D. Research)
My Ph.D. work at McGill University focused on the peculiar all-optical patterning known to occur in the azobenzene-polymer system. In 1995, it was discovered that the free surface of azo-polymer thin films would spontaneously deform in response to light gradients. In essence, the material is forming a surface hologram that reproduces any impinging light pattern. This single-step, all-optical patterning/lithography is fast, efficient, anI first wrote a cellular automata computer model to analyze the temperature distribution during laser inscription. This work allowed me to exclude thermal models as possible explanations for the mass-transport phenomenon. I also measured the thermal erasure of photo-induced surface features, using a variety of techniques from combinatorial material science. These experiments showed conclusively that the mass-transport requires coordinated polymer motion over long length-scales (~150 nm) and not merely molecular diffusion. Finally, neutron reflectivity studies were used to identify photo-mechanical effects in this system. It was found that light can be used to photo-expand and photo-contract the azo material. These photo-mechanical effects appear to be the origin for the unique mass transport.